Mapping a Tiny Mind
The age of neural cartography has arrived. And the result is visually stunning.
FlyWire, a collaboration between Mala Murthy and Sebastian Seung’s labs at Princeton University and numerous other researchers from a variety of institutions, charts the brain of a fruit fly in exquisite detail—mapping a staggering 139,255 neurons and the 54.5 million synapses connecting them.
The brain of a fruit fly may be smaller than a mustard seed, but it’s a dense tangle of processing power. Fit into that tiny package is the material needed to manage a tiny but complex organism that must fly, find food, escape from predators, and attract a mate.
This decade-long project relied on people and AI to create a 3D map of the fruit fly brain. "There’s neurons involved in navigation and heading,” says Amy Sterling, a neuroscientist at Princeton University and the crowdsourcing manager for FlyWire. “Different neurons are involved in taste and chemoreception,” she says. “There’s learning and memory. There’s actually a lot of basic decision making in fly brains.”
The findings were published in the fall to great fanfare, but most of the press coverage hasn’t explored the full array of visuals produced by the project. Here we'll do just that.
“It shows how highly connected the fly brain is,” says Sterling. “It is transformative for neuroscience.” This is the largest whole brain that’s ever been fully mapped, and it’s allowing scientists to ask—and in some cases, answer—a stunning array of new questions.
To understand what a breakthrough this is, Sterling says you really have to explore the map yourself. “When do you read about a map? It’s like reading a play instead of watching it,” she says.
The map began a decade or so ago when a team of researchers spent three weeks sectioning the brain of an adult female fruit fly into 7,062 extremely thin slices. Each slice was then scanned with an electron microscope and digitized. Moving through these slices from the front of the brain to the back, different structures enter into view.
First, we see the antenna lobes. “This is where olfactory information comes in,” says Philipp Schlegel, a neuroscientist at the University of Cambridge and one of the FlyWire project leads. “Then we see the optic lobes, on the left and on the right, slowly, surely appearing. And if I keep going, this doughnut shape in the middle, that’s the central complex. That’s the center for learning and memory.”
He selects one of the middle slices and zooms in on a region of the brain called the lateral horn, which is involved in hardwired innate behavior. There are two basic parts to the image:
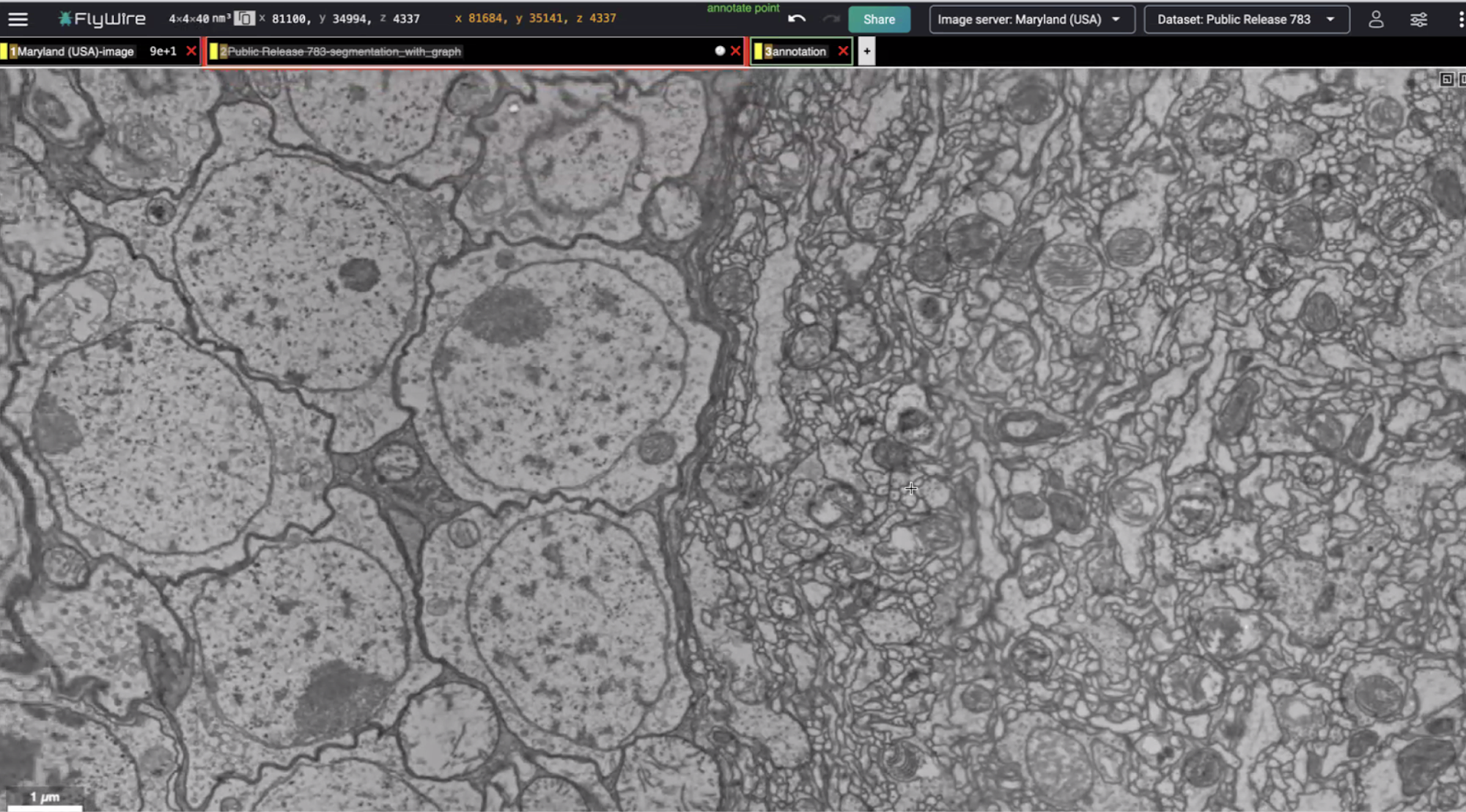
On the left, there's a collection of large cell bodies, each one belonging to a different neuron. These are where most of the nerve cells’ proteins are made.
On the right is something called the neuropil, a dense interwoven matrix of nerve fibers, neurotransmitters, and other cells. “This is where the computation takes place,” says Schlegel, and where nerve cells typically communicate. “The thing that actually makes the brain tick, at least for insect neurons.”
When the two are examined side by side like this, their appearance is “just day and night,” Schlegel observes. They look like separate entities, but each cell body has a projection that travels into the neuropil. In other words, a single nerve cell spans both sides of this image, containing a cell body and material in the neuropil. It’s not easy to tell by looking at a single slice like this. Instead, you have to engage with the brain in three dimensions.
This is where FlyWire enters the picture. First, Schlegel selects one of these cell bodies and traces its location in each successive slice. He snakes his way through the cell body’s projection as it travels a short distance before burrowing into the neuropil. “It’s almost like highways that crisscross through the brain,” says Schlegel.
Now, if we take this painted neuron and layer the slices into a 3-D representation, we get a single neuron unfurled in its corner of the brain:
“And this is a small neuron,” admits Schlegel.
Sterling eagerly calls up one of the largest brain cells that the team mapped, called CT1. “It’s a very large inhibitory neuron in the visual system,” she says. “It has this really crazy structure.”
At the other extreme, there are “some really teeny tiny ones,” says Sterling, that only talk to a handful of other cells. “There’s a huge variety in neuron size and complexity.”
Schlegel loads more examples of what he refers to as “the zoo of neurons.” Over vast parts of the brain, “no two neurons look exactly alike,” he says.
There are cells that are reminiscent of fireworks. Others resemble Spanish moss. The longest neuron in the fruit fly brain with over 100,000 synapses looks like a wiry boomerang.
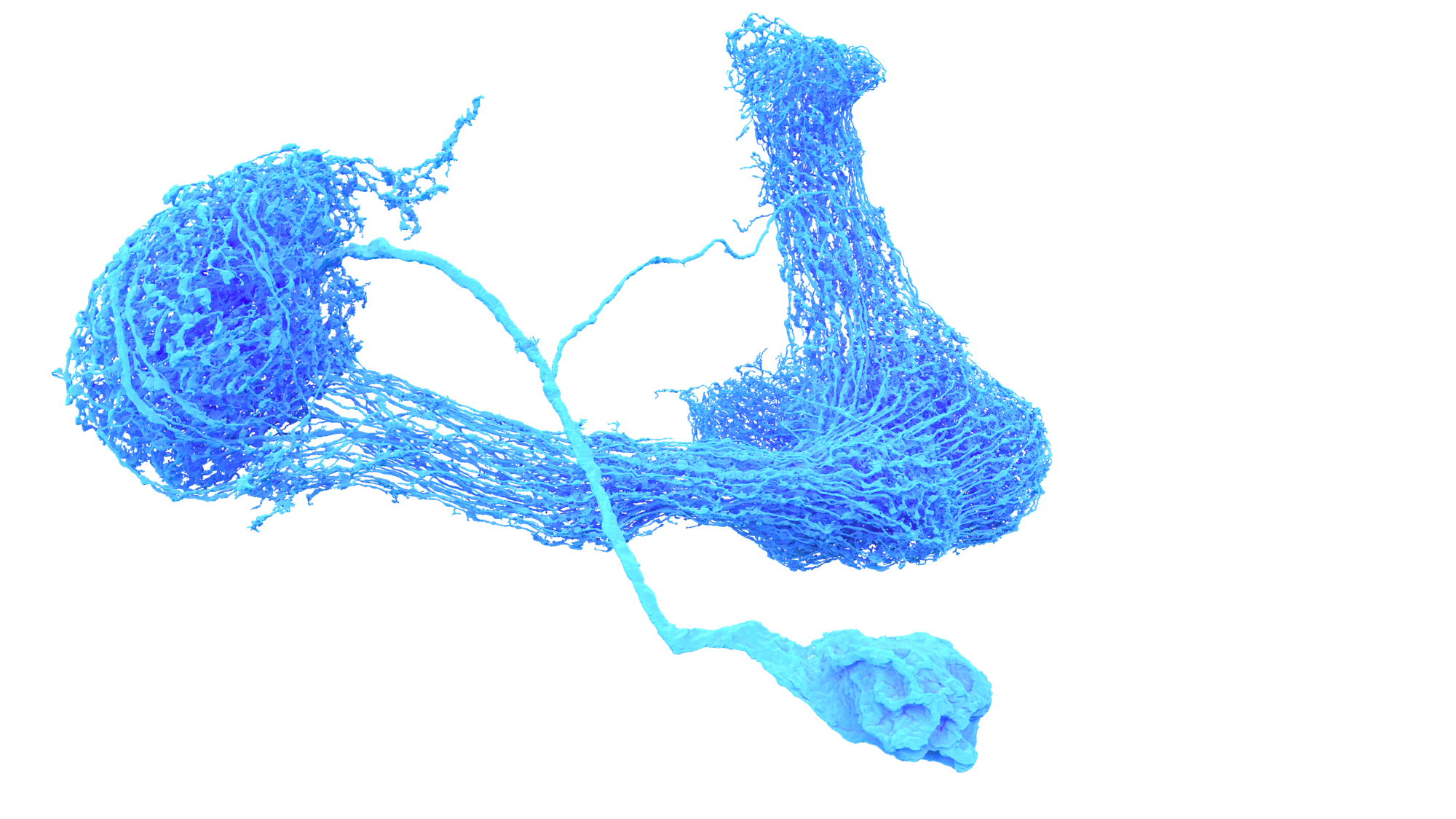
The brain’s cells were initially mapped using automated AI approaches. “But the computer still makes mistakes,” says Schlegel, “and those have to be fixed up by humans” one at a time. If you were to add all the time spent by everyone working on the project, the scientists estimate the reconstruction of the entire fly brain took years of manual proofreading.
Of course, neurons don’t exist in isolation. They’re interconnected, like a three-dimensional tapestry. CT1 has 55,648 incoming connections or synapses with 4,780 cells and 92,957 outgoing synapses with 6,211 cells. But that’s a Sasquatch. The average fruit fly neuron, says Schlegel, “will be much smaller and have synaptic inputs in the range of a few hundred.”
Here’s another example. Take this neuron, a cell that receives information from the antennae and passes it along to higher brain centers.
Schlegel toggles on the neurons that originate in the antennae and target this cell. Here they are, draped across the original neuron like a ball of colorful yarn unfurling:
This bundle of cells may respond to a specific scent like a pheromone, or to a broader class of aromas, like flowers. Computation then happens inside this snarl of color — signal processing and sharpening, noise reduction, and more — before it relays the information to a part of the brain that can make sense of it and influence the fly’s behavior.
Sterling calls up one of her favorite parts of the brain—the ring neurons of the ellipsoid body, which are involved in navigation. “The different directions of a compass are basically encoded in here,” she says. “They’re color coded by the heading.”
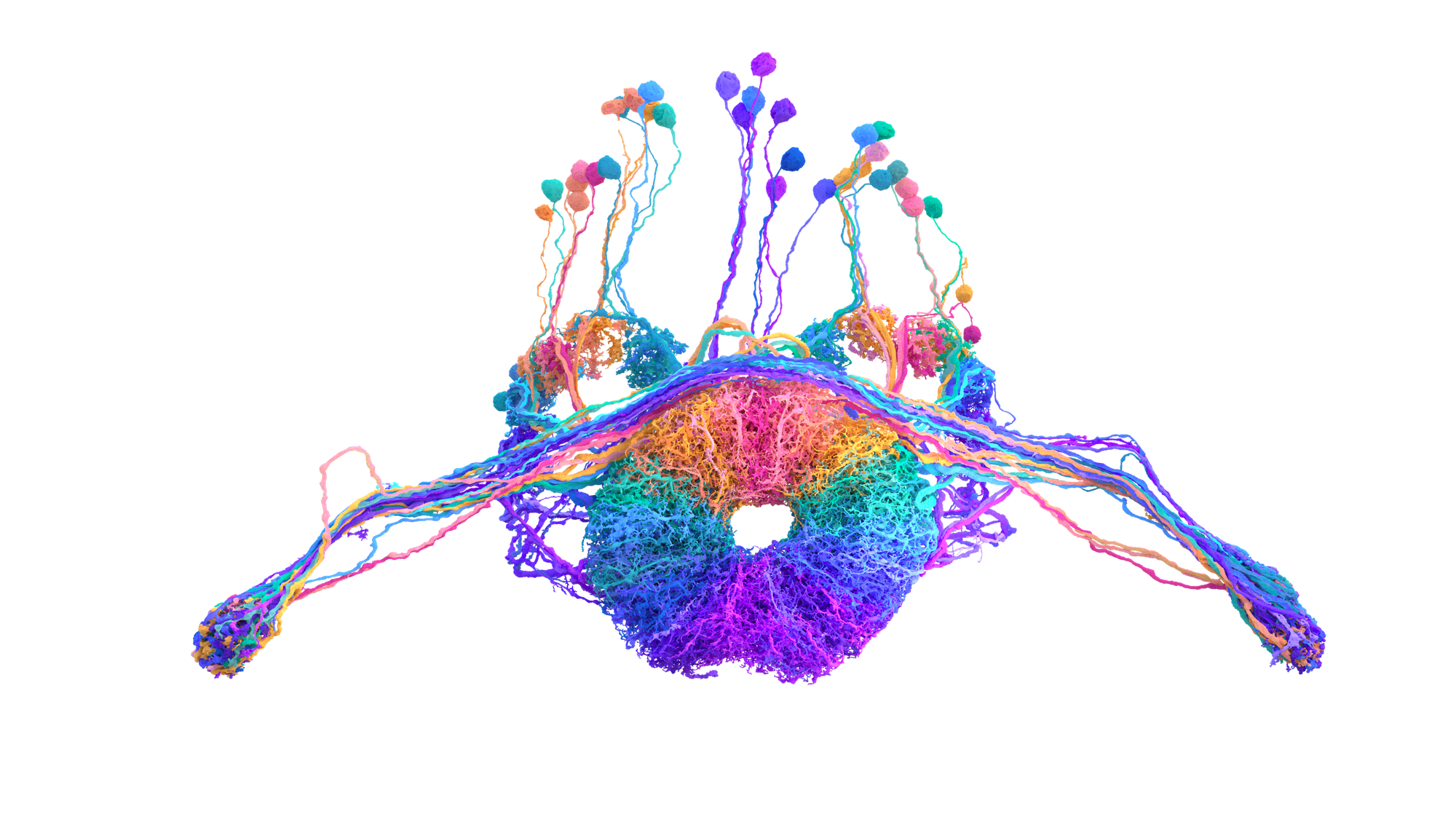
Another way of visualizing parts of the brain is according to function. If we consider all the visual neurons at once, it’s evident just how much real estate the fruit fly devotes to vision:
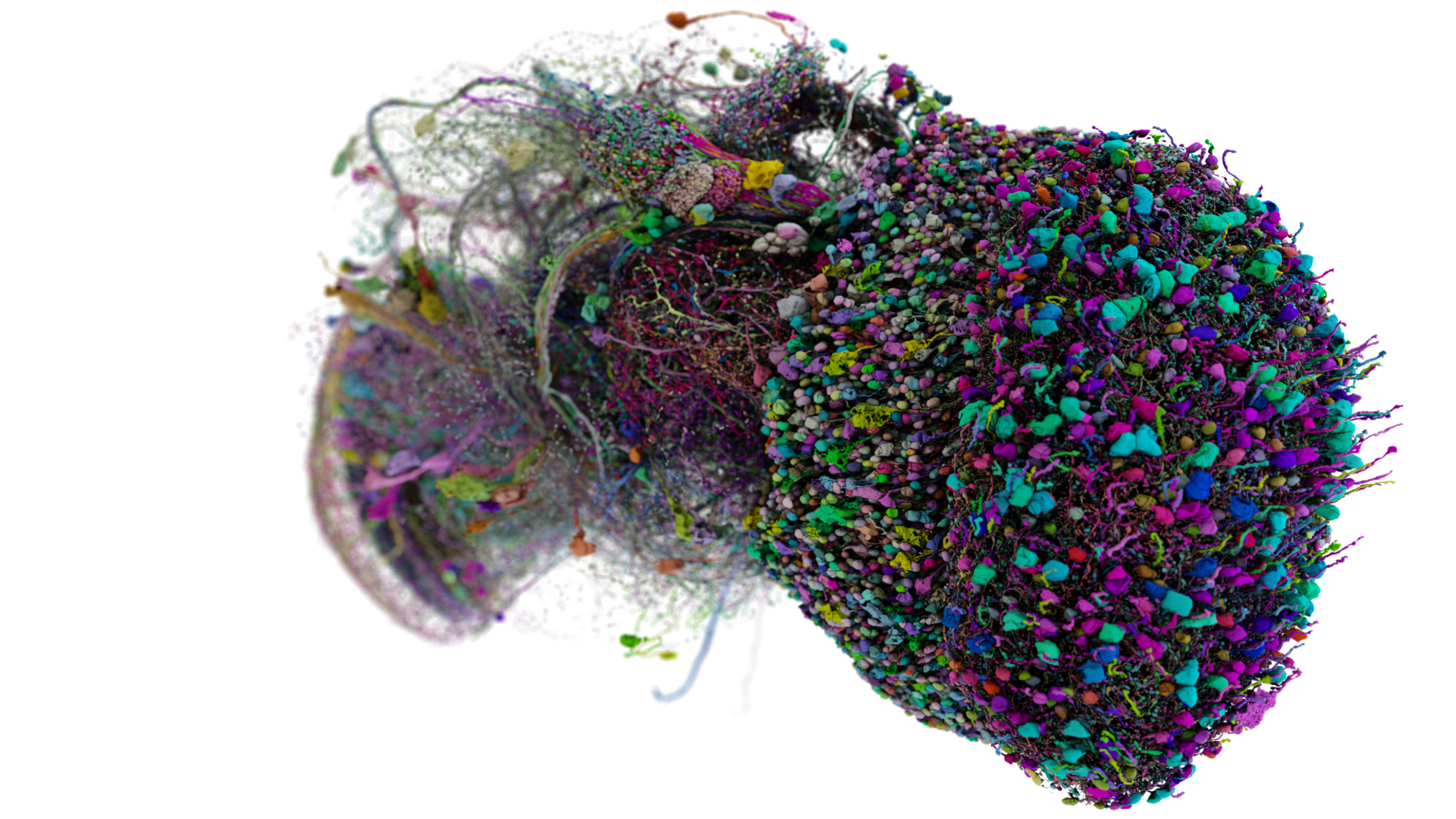
The dizzying array of connections between neurons, mapped across the entire fly brain, is known as the connectome.
“Now it’s just a totally different world,” Schlegel says. “I can do four years’ worth of a PhD now by browsing this for ten minutes. That’s the kind of step change that we’re looking at.”
Sterling helped design Codex, the tool that allows anyone to explore the connectome through a user-friendly interface. “Prior to Codex, the only way to explore the data was with code,” she says. But now, you can search for a particular cell and “see all of its up and downstream partners. You can visualize the pathways and network connectivity of neurons.”
Each neuron has its own page on Codex:
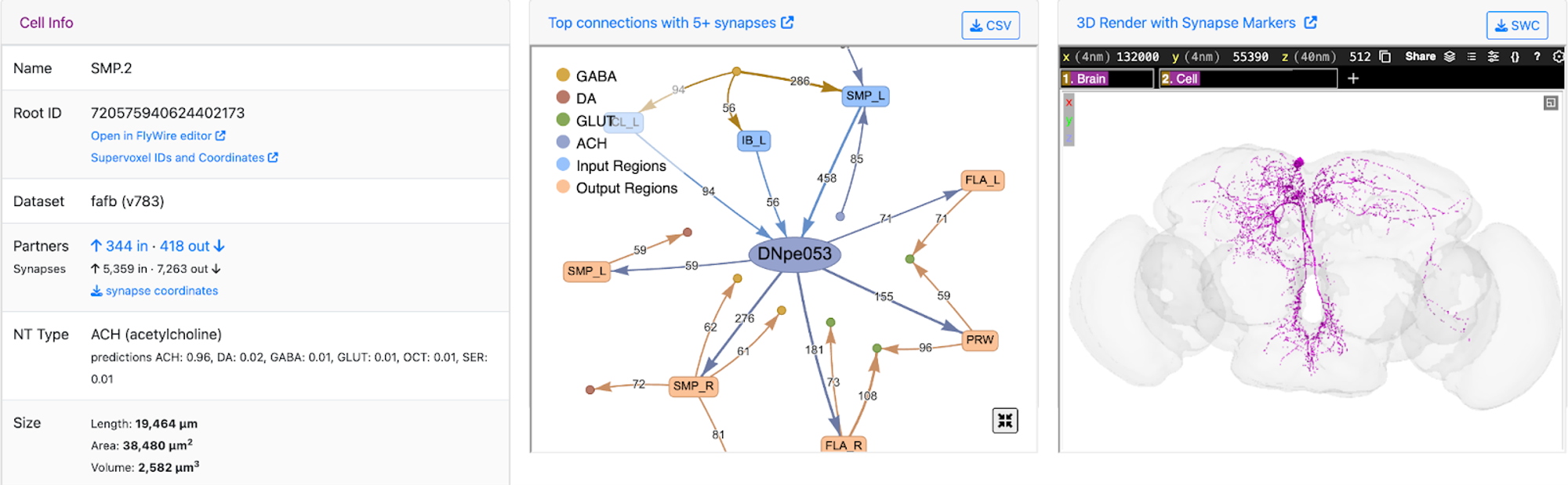
And once someone has created a visualization using Codex, Sterling says you can just copy the link and share it with anyone, anywhere.
She sees FlyWire as an accelerant for neuroscience. “It’s not like everyone is having to do a Lewis-and-Clark-style traverse across the whole country to find what’s on the other side,” she says. “People can just look at the map and figure out how the brain is controlling behavior [and] what role specific neurons or circuits have in behavior. And so having this whole [map of the] brain network enables that in a way that’s never before been possible.”
Once a researcher identifies a neuron of interest, Sterling says they can use the connectome to map its circuitry within the brain “and then link that circuitry to behavior.” With genomics, it’s then possible to understand the role a particular gene has on that neuron, circuit and behavior.
Because fruit flies share many of their genes with other species, including humans, there are medical applications to this work. Schlegel explains the fruit fly is used as a model to study diseases like Parkinson’s and Alzheimer’s.
But he’s more interested in how the structure and wiring of such a tiny speck of nervous tissue, built and refined over millions of years of evolution, can now do so much—how the fly does all it can “on hardly any hardware.” Such insights will inform the field of neuromorphic computing, which uses the architecture of brains to inspire how to build and operate a computer.
Schlegel is excited to see what emerges from the new dataset, especially as it’s paired with new experiments.
When the researchers mapped those thousands of neurons together and linked them up with millions of synapses, the result was the very thing that makes a fruit fly the buzzing wonder that it is. “It just goes to show how beautiful and incredibly detailed that data is when you actually get the chance to look at it up close,” says Schlegel.
All of the FlyWire data is publicly available and fully interactive—something that doesn’t always happen in science, which can be quite competitive. “We were pretty adamant about making it open and collaborative the whole time,” says Sterling. “Hopefully, this can expedite progress across different scientific disciplines.”
Schlegel says this map serves as a gateway for neuroscientists to design experiments that will deepen our understanding of how brains work more generally. And the tools and approaches that were developed to map this speck of a brain are already being put to use in mapping an even larger brain—that of the mouse, which has 1,000 times more neurons than the crackling bundle of thought, behavior and instinct of the fruit fly. ♦
Subscribe to Broadcast